Research
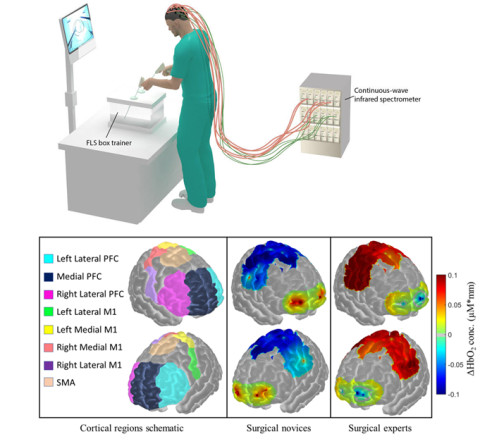
What defines an expert surgeon? Does a surgical expert have finer motor skills than a resident or novice? Can this be objectively measured to help measure surgical performance? The answer to these questions can help reduce over 300,000 perioperative mortalities that cost over $9 billion each year. Unfortunately, current metrics to measure surgical performance are subjective and cannot robustly differentiate between surgical novices or experts, thus prompting for more objective and analytical approaches.
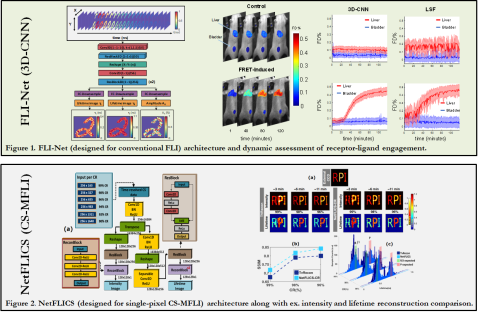
Deep learning continuously offers improvements over conventional techniques in biosignal processing and computer vision – such as enhanced analytic speed, lower user-dependent bias and robustness to noise. Our lab has recently developed and heavily validated unique analytic workflows based on deep learning to tackle the inverse solving process inherent to our bioimaging technologies - such as complex FLI (microscopically and macroscopically), diffuse optical tomography, and fNIRS - across multiple challenging applications and technologies. By using state-of-the-art Monte Carlo modeling of light propagation, experimentally representative data is simulated over the ranges & conditions expected in biological application and used for training deep neural networks (DNNs). The improved reconstruction qualities observed, as well as the real-time employability achieved, is a huge step towards high-throughput pre-clinical & clinical application.
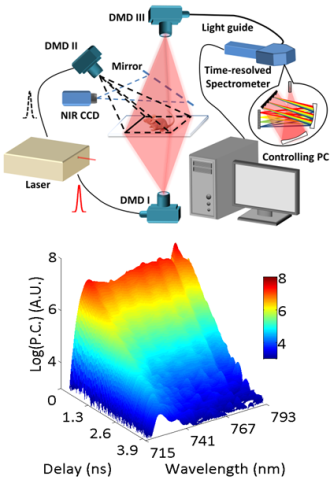
Diffuse Optical Imaging (DOI) enables non-invasive probe of optical contrasts (absorption, fluorescence intensity and lifetime) in 2D and 3D turbid media for structural, functional and molecular information. Time-resolved DOI (TD-DOI) is widely employed to monitor tissue microenvironment (through pH value, local temperature, local glucose concentration, tissue hemodynamics and so on) as well as protein-protein interactions via Förster Resonance Energy Transfer (FRET). Single-pixel imaging is an emerging technique which allows for the multiplexing and reconstruction of spatial, temporal and spectral information with highly-compressed data sets and improved Signal-to-Noise Ratio (SNR).
We proposed a platform to perform hyperspectral single-pixel TD-DOI and validate its performance with in vitro and in vivo, 2D planar and 3D tomographic studies. The system employs a Mai Tai high power pulsed laser as light source. Two DMD (Digital Micro-mirror Device)-based spatial light modulators form two illumination channels: reflectance and transmission, and a third DMD forms the detection channel with a multi-spectral time-resolved Photo Multiplier Tube (PMT). Structural optical masks are applied in both illumination and detection sides, so that dense spatial, temporal and spectral information from the sample can be acquired simultaneously.
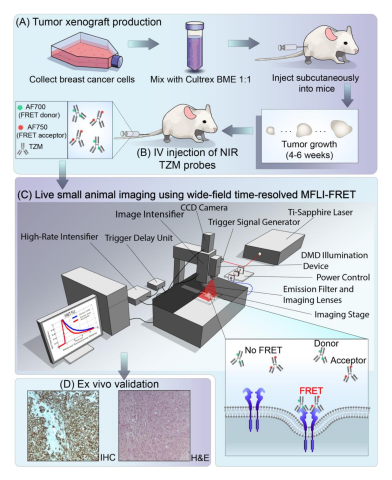
Optical imaging (OI) can be used as a non-invasive tool for assessment of drug delivery. Most modalities are intensity-based, so cannot discriminate between extracellular accumulation and intracellular delivery. Förster Resonance Energy Transfer (FRET), oftentimes referred to as the "molecular ruler", is a physical phenomenon involving two fluorophores – donor and acceptor. Measuring FRET enables one to assess molecular interactions on the nanoscale, such as quantifying intracellular delivery, across large fields of view with short acquisition times.
There are two approaches in FRET quantification – intensity-based and lifetime-based. Lifetime FRET sensing is non-invasive and non-radiative approach. Förster Resonance Energy Transfer (FRET) is the radiationless transfer of energy from an excited donor fluorophore to an appropriate acceptor in close proximity. The energy transfer only occurs between fluorophores separated by less than ~10 nm, allowing to sense protein interactions. However, to date, FRET applications are confined to microscopy or spectroscopy of cells culture or cell lysates. It is critical to translate FRET assays to small animal imaging where the in vivo physiological context is essential for drug development, the study of diseases, and fundamental cellular and molecular biology. We develop new methods to perform quantitative FRET tomography in live animals. Learn more>>

There is a major drive in Tissue Engineering towards creating functional tissues or organs in vitro in 3D thick scaffolds. Such scaffolds are generally thick (few mm) and constructed using biomaterials that are highly-scattering (such as elastin or collagen). Hence, laser scanning microscopy techniques (e.g., confocal and two-photon) cannot image entirely these scaffolds as the depth penetration of such techniques is still limited by the scattering properties of the sample. Thus, there is a critical need to develop new imaging techniques that can image well beyond the penetration limits of conventional microscopy. We develop novel multispectral mesoscopic fluorescence molecular imaging platform to this end.
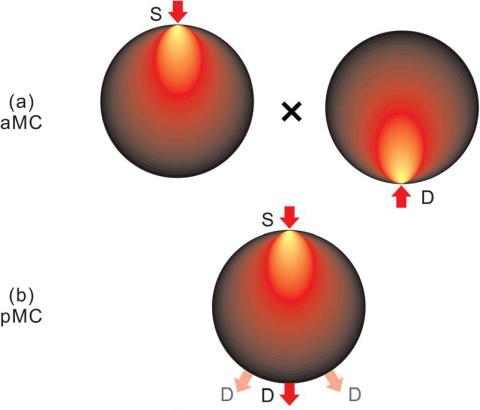
To perform quantitative tomography, it is essential to employ an accurate mathematical model to describe the propagation of light in biologic tissues. The Monte Carlo method is a statistical method used to simulate and track individual photons as they propagate. This method is considered the gold standard to accurately model light propagation in either diffusing or non-diffusing media with flexibility to model arbitrary boundaries. We develop massively parallel computational platforms that allow computing time-resolved Jacobians with the pMC, the adjoint method (aMC) and/or the midway adjoint method (mMC).
We are pioneering wide-field optical tomography based on time-resolved patterned illumination for preclinical studies. Such innovative approach allows to acquire dense spatial and temporal tomographic data-sets over the animal whole-body at unprecedented acquisition speeds ( <10min from neck to tail, full temporal data). In our instrumental approach, we have implemented a wide-field excitation scheme based on micromirror technology to generate time-resolved illumination patterns. This new approach offers numerous advantages compared to current excitation scheme: i) exceptionally fast acquisition of spatially dense tomographic information over large volumes; ii) injection of a greater number of photons into the overall tissue leading to measurements in transmittance with higher SNR, especially in fluorescence applications (greater sensitivity to minute fluorophore concentrations) and iii) higher number of useful detector measurements for pattern due to spatially extended sources; iv) faster reconstructions (forward calculation and inversion) through the use of smaller weight matrices (owing to lower number of sources being used). Wide-field optical tomography allows for quantitative functional imaging and lifetime multiplexing studies.